1. Introduction
In the quest for sustainable energy solutions, significant strides have been made in the fields of Proton Exchange Membrane Fuel Cell (PEMFC), catalyst, ink formulation and water electrolyzer. These technologies are crucial for the transition to renewable energy, offering efficient, clean, and scalable options for energy production and storage. This article delves into the intricate details of these advancements, exploring their background, purpose, experimental methods, and future prospects.
2. Proton Exchange Membrane Fuel Cell
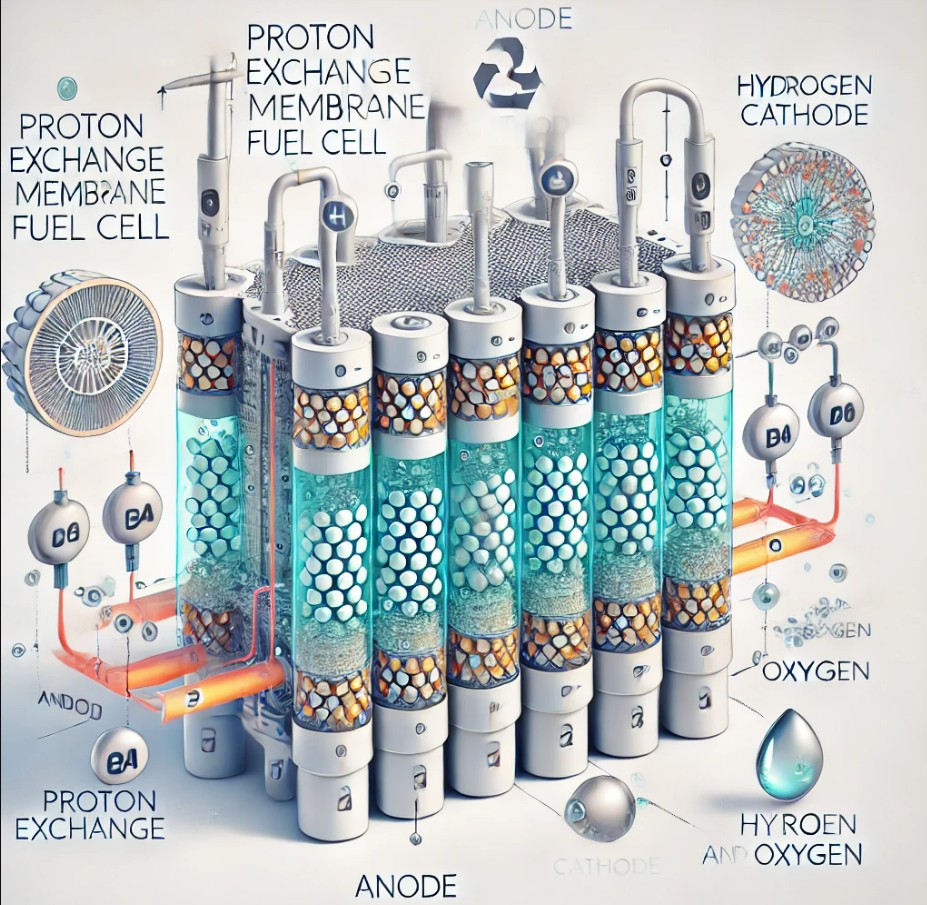
2.1 Overview of PEMFC Technology
Proton Exchange Membrane Fuel Cell (PEMFC) is a type of fuel cell that converts chemical energy from hydrogen into electrical energy through an electrochemical reaction. This process involves the oxidation of hydrogen at the anode and the reduction of oxygen at the cathode, with a proton-conducting membrane facilitating the transfer of protons. PEMFC operates at relatively low temperatures and are known for their quick startup and high power density.
2.2 Advantages and Applications
Proton Exchange Membrane Fuel Cell is favored for their high efficiency, low operating temperatures, and rapid startup times. They are used in various applications, including transportation (fuel cell vehicles), stationary power generation, and portable power devices. The shift towards PEMFC is driven by the need for cleaner energy alternatives to reduce carbon footprints.
2.3 Background and Purpose
The development of Proton Exchange Membrane Fuel Cell has been driven by the need to reduce dependence on fossil fuels and decrease greenhouse gas emissions. The purpose of advancing PEMFC technology is to create more efficient, cost-effective, and durable systems that can be widely adopted in various sectors. Research focuses on improving the performance of the fuel cell components, particularly the catalyst layers and membranes.
3. Water Electrolyzer
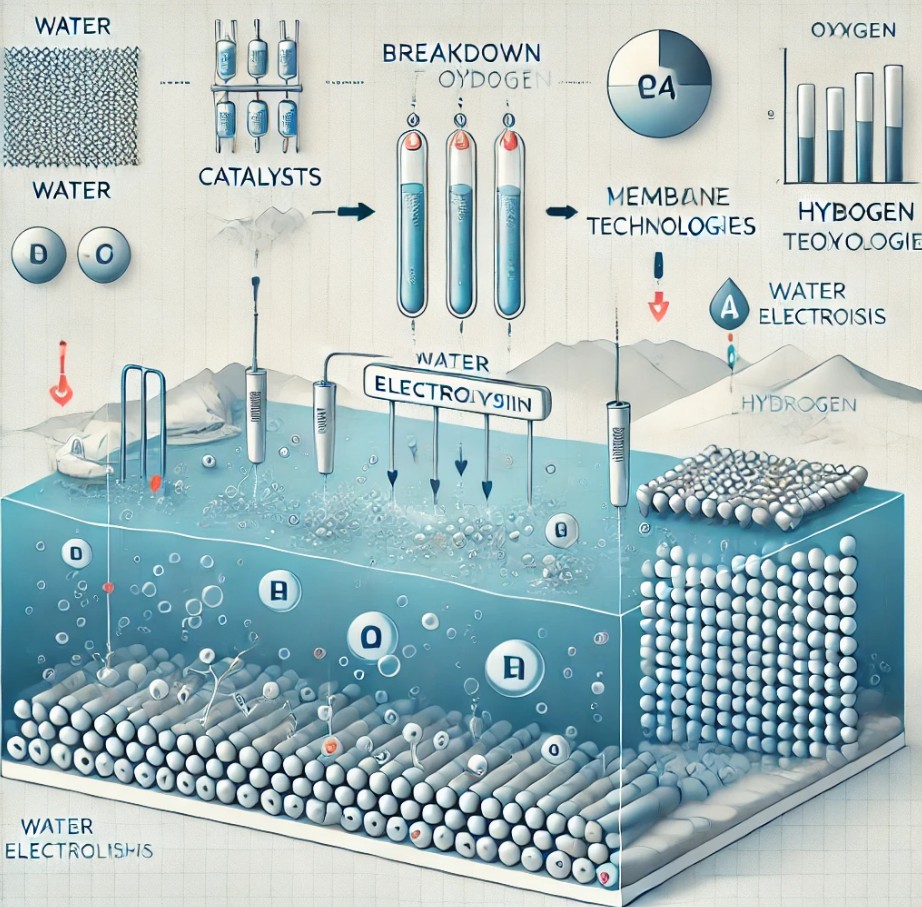
3.1 Role in Hydrogen Production
Water electrolyzer(PEMWE) is vital for hydrogen production, splitting water into hydrogen and oxygen using electrical energy. PEMWE offer high efficiency and purity of hydrogen, making them suitable for various industrial applications.
3.2 Technological Advancements
Technological advancements in water electrolyzer focus on improving the efficiency and reducing the costs of hydrogen production. Innovations in catalyst materials and membrane technologies are at the forefront of this research.
3.3 Background and Purpose
The push for advancements in water electrolyzer stems from the increasing demand for clean hydrogen as an energy carrier. The purpose of these advancements is to make hydrogen production more economical and scalable. Research focuses on improving the catalytic efficiency and durability of the electrolyzer components, as well as enhancing the overall system design.
4. Catalysts in PEMFC and Water Electrolyzer
4.1 Importance of Catalyst
Catalyst are essential in both Proton Exchange Membrane Fuel Cell and water electrolyzer, as they facilitate the electrochemical reactions at the electrodes. The choice of catalyst directly impacts the efficiency, durability, and cost of these systems. Platinum-based catalysts are commonly used in PEMFC for their excellent activity and stability, while iridium-based catalysts are preferred for water electrolyzer due to their high efficiency in oxygen evolution reactions.
4.2 Recent Advances
Recent research has focused on developing more efficient and cost-effective catalysts. Innovations include the use of alloyed catalysts, core-shell structures, and alternative materials such as transition metal carbides and nitrides. These advancements aim to reduce the reliance on precious metals and enhance the overall performance of the fuel cell and electrolyzer.
4.3 Background and Experimental Methods
The background research on catalysts involves understanding the fundamental mechanisms of the electrochemical reactions and the role of different materials in these processes. Experimental methods include synthesis techniques such as chemical vapor deposition, atomic layer deposition, and solvothermal methods. Characterization techniques like X-ray diffraction (XRD), transmission electron microscopy (TEM), and cyclic voltammetry (CV) are used to study the structural and electrochemical properties of the catalysts.
5. Catalyst Ink Formulation
5.1 Composition and Properties
Catalyst ink formulations are critical in determining the performance of PEMFC and electrolyzer. These inks typically consist of supported catalysts, ionomers, and solvents. The formulation affects properties such as homogeneity, interaction between components, storage stability, and reproducibility.
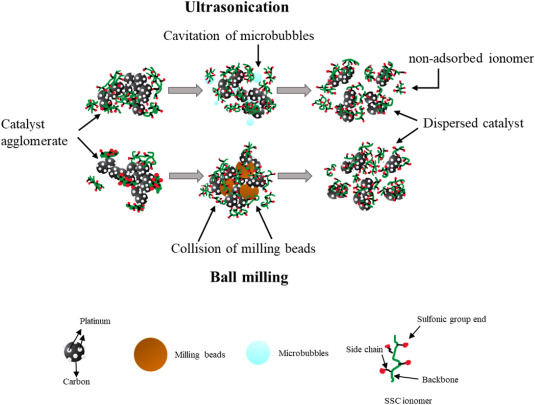
5.2 Factors Influencing Ink Stability
Ink stability is influenced by factors like particle surface properties, solvent composition, and ionomer concentration. Stable inks ensure consistent performance and are crucial for large-scale manufacturing. Understanding the colloidal stability and optimizing the interaction between components can lead to better-performing catalyst layers.
5.3 Background and Experimental Methods
The development of catalyst inks involves extensive research into the interaction between the ink components. The background includes studies on the dispersion stability, rheological properties, and the effects of different solvents and ionomers. Experimental methods include dynamic light scattering (DLS) for particle size analysis, zeta potential measurements for surface charge assessment, and rheology for understanding viscosity and flow behavior. Techniques such as ultrasonication, ball milling, and high-shear mixing are employed to achieve uniform dispersions and stable ink formulations.
6. Characterization and Optimization Techniques
6.1 Characterization Tools
Characterizing the properties of catalyst inks and layers involves techniques like Dynamic Light Scattering (DLS) for particle size analysis, zeta potential measurements for surface charge assessment, and rheology for understanding viscosity and flow behavior.
6.2 Optimization Strategies
Optimizing the formulation and processing of catalyst inks can significantly enhance the performance of PEMFC and electrolyzer. Techniques such as ultrasonication, ball milling, and high-shear mixing are employed to achieve uniform dispersions and stable ink formulations.
6.3 Background and Experimental Methods
The background research for characterization and optimization involves studying the effects of different formulation parameters on the stability and performance of the catalyst inks. Experimental methods include advanced microscopy techniques like scanning electron microscopy (SEM) and atomic force microscopy (AFM) to analyze the microstructure of the catalyst layers. Spectroscopic methods such as X-ray photoelectron spectroscopy (XPS) are used to study the chemical composition and surface properties.
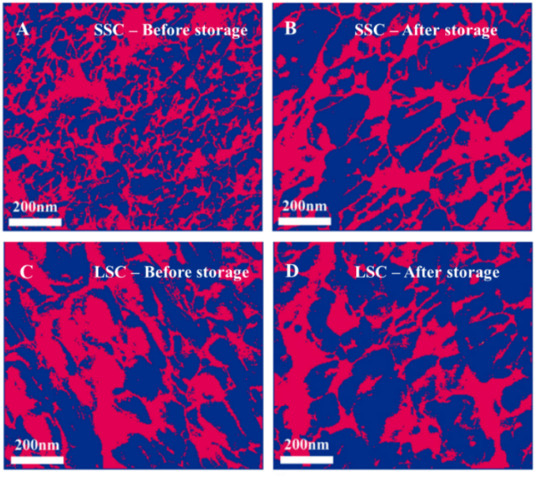
7. Future Directions and Prospects
7.1 Research and Development
Ongoing research aims to further improve the efficiency, durability, and cost-effectiveness of PEMFC, catalysts, and water electrolyzer. The focus is on developing new materials, enhancing ink formulations, and scaling up manufacturing processes.
7.2 Industrial and Environmental Impact
The advancements in these technologies have the potential to revolutionize the energy sector, providing cleaner alternatives to fossil fuels. The widespread adoption of PEMFC and electrolyzer can significantly reduce greenhouse gas emissions and contribute to a sustainable energy future.
7.3 Background and Purpose
The future research direction is shaped by the need to address the limitations of current technologies and to meet the increasing demand for clean energy solutions. The purpose is to achieve breakthroughs in material science, engineering processes, and system integration to make these technologies more accessible and efficient. Collaborative efforts between academia, industry, and government agencies are crucial to drive innovation and implementation.
8. Conclusion
The progress in PEMFC, catalyst, ink formulation and water electrolyzer marks a significant step towards sustainable energy solutions. By understanding and optimizing these components, we can unlock the full potential of hydrogen as a clean energy carrier, paving the way for a greener future.
Source: Murugaiah, Dhinesh Kumar, and Samaneh Shahgaldi. “Recent progress in understanding the dispersion stability of catalyst ink for proton exchange membrane fuel cell and water electrolyzer.” International Journal of Hydrogen Energy 66 (2024): 156-169.
https://doi.org/10.1016/j.ijhydene.2024.04.036
※ This report has been compiled for the purpose of providing general information. It is based on data gathered by CHEMiFORGE. Should you have any inquiries or need to make decisions based on this report, it is advisable to consult with a CHEMiFORGE.